Correlation between Kelch13 mutations and clinical phenotype Study Group - update
Correlation between Kelch13 mutations and clinical phenotype Study Group - update
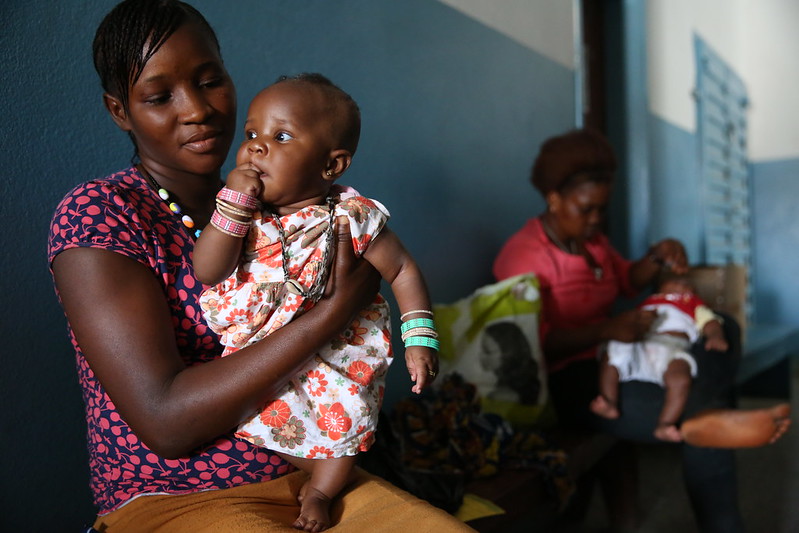
The Study Group was formed in January 2024 to update and expand the WWARN work of the K13 Genotype-Phenotype Study Group, which was published in BMC Medicine (January 17, 2019) on the Association of mutations in the Plasmodium falciparum Kelch13 gene (Pf3D7_1343700) with parasite clearance rates after artemisinin-based treatments—a WWARN individual patient data meta-analysis. Since then, artemisinin partial resistance has been confirmed in a rapidly increasing number of countries outside of South-East Asia, including in sub-Saharan Africa, where the vast majority of malaria cases and deaths occur. The ramifications of artemisinin (partial) resistance (ART-R) are now understood to extend beyond delayed parasite clearance and include greater pressure on artemisinin partner drugs, subsequently increasing the risks of artemisinin-based combination therapy treatment failure.
Artemisinin-based combination therapies (ACTs) are currently the only recommended drugs to treat uncomplicated and severe Plasmodium falciparum malaria and have contributed substantially to the dramatic decrease in malaria incidence and mortality since 2000. However, the emergence of P. falciparum ART-R in and beyond South-East Asia, particularly in high-burden areas in sub-Saharan Africa, threatens to jeopardise those gains (Rosenthal et al., 2022).
ART-R has been associated with delayed parasite clearance after ACTs or artemisinin monotherapy and a number of mutations in the propeller region of the K13 gene (Dondorp et al., 2009; Ariey et al. 2014; Ashley et al., 2014WWARN K13 Genotype-Phenotype Study Group 2019). The subsequent spread and evolution of ART-R, coupled with the acquisition of resistance to ACT partner drugs, have led to high rates of ACT treatment failure in Southeast Asia (Dhorda et al., 2021; Peto et al., 2022, Spring et al, 2015). Although resistance to artemisinins and partner drugs poses a significant threat to the efficacy of first-line ACTs, its impact in Southeast Asia has been tempered by the relatively low malaria burden and remarkable investments in improved malaria control and elimination in this region (WHO, 2022a). However, in sub-Saharan Africa, which suffers over 90% of the world’s malaria burden, progress in malaria control has stalled since 2015, with gains achieved since the turn of the century partially reversed during the COVID-19 pandemic. In this context, recent reports of the de novo emergence and spread of ART-R in Africa (Ndwiga et al., 2021; Rosenthal et al., 2024), particularly in East Africa (Asua et al., 2019; Uwimana et al., 2020; Uwimana et al., 2021; Balikagala et al., 2021; Bergmann et al., 2021; Moser et al., 2021; Owoloye et al, 2021; Straimer et al., 2022; Conrad et al, 2023) and the Horn of Africa (Mohamed et al., 2020; de la Fuente et al., 2023; Emiru et al., Fola et al., 2023; 2023; Jalei et al., 2023; Mihreteab et al., 2023;) is of enormous concern (Rosenthal et al., 2022; WHO 2022b; Assefa et al., 2024).
There is limited evidence that K13 mutations may have been associated with loss of ACT treatment efficacy in Africa. A number of African countries with confirmed artemisinin resistance have recently reported PCR-corrected efficacy below the 90% WHO-recommended threshold of acceptability (WHO Malaria Threat Maps). However, factors other than drug resistance, such as varied study methods, may influence ACT therapeutic efficacy study results. In Southeast Asia, the rise in artemisinin resistance was soon followed by the emergence and spread of resistance to ACT partner drugs and frequent ACT treatment failures (Amaratunga et al., 2016; Phyo et al., 2016; Phuc et al., 2017; van der Pluijm et al., 2019). A similar trajectory in sub-Saharan Africa - in particular with the loss of lumefantrine, the most widely used ACT partner drug and a component of the two novel antimalarials currently in Phase 3 clinical trials (MMV 2024) - would jeopardise the treatment of hundreds of millions of patients each year, likely leading to marked increases in malaria morbidity and mortality (Rosenthal et al, 2022).
Molecular surveillance alone has identified K13 mutants in many sites (WHO Malaria Threat Map), but without a correlation of these alleles with a clinical phenotype, their public health significance is not well understood, particularly in sub-Saharan Africa. A number of studies assessing the relationship between the K13 molecular markers of resistance and delayed parasite clearance and ACT therapeutic efficacy have been published recently. Unfortunately limited evidence is currently available on the K13 molecular markers of resistance and malaria transmission (e.g. gametocytes, membrane feeding experiments). Thus, this IPD meta-analysis of collated published and unpublished studies will explore the relationships between K13 mutant alleles and artemisinin-based treatment response – parasite clearance and therapeutic efficacy; a subsequent IPD meta-analysis will assess the effect on malaria transmission (data permitting).
To assess the global prevalence, distribution, and functional significance of pfK13 propeller mutations and treatment response
- To define the global prevalence, distribution and functional significance of pfK13 propeller mutations in terms of:
- Parasite clearance half-life (PC1/2) in patients with falciparum malaria treated with artemisinin-based combination therapies (ACTs) or monotherapy in antimalarial clinical studies by location, treatment, study population and date.
- Parasite positivity on Day 2 and Day 3 in patients with falciparum malaria treated with artemisinin-based combination therapies (ACTs) or monotherapy in antimalarial clinical studies by location, treatment, study population and date.
- Clinical and parasitological treatment response in patients with falciparum malaria treated with artemisinin-based combination therapies (ACTs) or monotherapy in antimalarial clinical studies by location, treatment, study population and date.
2. To compare results between different geographical regions, such as Asia and Africa, and intra-regional comparisons, such as Eastern and Southern Africa.
3. To investigate spatial-temporal differences in the relationship between delayed parasite clearance and K13 alleles from recent data in comparison to the conclusions drawn from the previous investigation (WWARN et al. 2019)
Results of kelch13 genotyping (number of isolates genotyped, number of each pfk13 WT or mutant codon) at baseline (Day 0 preferred; otherwise, earliest day up until Day 3).
AND
Prospective clinical trials in patients with uncomplicated falciparum malaria with at least one study arm receiving treatment with any artemisinin-based combination therapy or artemisinin monotherapy, AND at least one of the following endpoints measured:
- Parasitaemia measured at least twice daily until the first negative blood smear; and / OR
- Parasitaemia measured on day 2 and/or 3; and / OR
- PCR-corrected therapeutic efficacy measured at day 28 and/or 42
Other inclusion criteria
- P. falciparum malaria (alone or mixed infection with other species) confirmed and asexual parasitaemia quantified by microscopy at enrolment and at the stated date / time of each subsequent measurement.
- Patient demographics (age, weight, gender)
- Information on dosing (mg/kg for artemisinin derivative, at least as per protocol)
Study meta-data
- Study location/s, study year/s, study population/s;
- Study design (e.g. randomised controlled trial, observational study);
- Artemisinin-based treatment/s and dosing strategies (age-based or weight-based; fat coadministration; supervised/partially supervised/unsupervised);
- Microscopy quantification methodology for asexual parasites
- Kelch13 genotyping methods;
- Transmission intensity (low / moderate / high) of each study site.
- Severe malaria on enrolment
Desirable additional information
- Molecular markers of artemisinin partner drug resistance;
- Patient height
- Presence of fever (body temperature > 37.5 degrees Celsius) at baseline;
- Any comorbidities;
- Any concurrent medication (e.g. rifampicin);
- Individual ACT or artemisinin monotherapy dosing data (mg/kg dose; actual dose times, treatment supervision; administration with food, any vomiting within 1 hour of dosing).
For Therapeutic efficacy studies:
- Methods for PCR genotyping to distinguish reinfection and recrudescence.
After uploading to the WWARN Data Repository, WWARN standardised clinical data sets according to the IDDO-SDTM Implementation Manual and pooled them into a single database of quality-assured individual patient data.
Individual patient data from selected studies published from 1995 to the present and meeting inclusion criteria will be used in this WWARN IPD meta-analysis.
The WWARN parasite clearance estimator tool (PCE) will determine the parasite clearance curve.
These pooled analyses will summarise all the included studies according to study location, years of study, study population, methodology for parasite quantification, genotyping methods, number of isolates genotyped, number of
pfk13 mutant codons, parasite clearances half-life and parasite clearances rate, day 2/3 parasite positivity, and PCR-corrected and uncorrected therapeutic efficacy on day 28+. A multivariable linear regression model with random effects for the study site will be used to investigate factors affecting treatment response. Covariates for the final regression models will be selected based on the likelihood ratio test and examination of residuals. To report a summary effect of all relevant studies in any model, we will compute the mixed effects analysis. Those parameters of heterogeneity will be presented graphically together by geographical region and transmission intensity.
The Study Group comprised participating investigators who contributed relevant data sets to the pooled analysis. Data sets remain the property of the investigator. The Study Group collectively made decisions regarding the inclusion of additional studies, data analysis, and plans for publication in line with the WWARN Publication Policy. The Study Group will include those who coordinate activities, including data analysis, draft publications, and reports for group review. The WWARN statistician(s) will be responsible for statistical analyses.
For further information, please contact Karen.Barnes@uct.ac.za
Amaratunga C et al., 2016. Dihydroartemisinin–piperaquine resistance in Plasmodium falciparum malaria in Cambodia: a multisite prospective cohort study. Lancet Infect Dis 16: 357–365. Ashley, E.A. et al., 2014. Spread of Artemisinin Resistance in Plasmodium falciparum Malaria. New England Journal of Medicine, 371(5), pp.411–423.
Ariey, F. et al., 2014. A molecular marker of artemisinin-resistant Plasmodium falciparum malaria. Nature, 505(7481), pp.50–5.
Ashley EA, et al. Spread of artemisinin resistance in Plasmodium falciparum malaria. N Engl J Med. 2014;371:411–23
Assefa, A., Fola, A.A. & Tasew, G. Emergence of Plasmodium falciparum strains with artemisinin partial resistance in East Africa and the Horn of Africa: is there a need to panic? Malar J 23, 34 (2024).
Asua V, Vinden J, Conrad MD, et al. Changing Molecular Markers of Antimalarial Drug Sensitivity across Uganda. Antimicrob Agents Chemother. 2019;63(3):e01818-18. Balikagala B, Fukuda N, Ikeda M, Katuro OT, Tachibana SI, Yamauchi M, et al. Evidence of artemisinin-resistant malaria in Africa. N Engl J Med. 2021;385:1163–71.
Bergmann C, van Loon W, Habarugira F, et al. Increase in Kelch 13 Polymorphisms in Plasmodium falciparum, Southern Rwanda. Emerg Infect Dis. 2021;27(1):294-296. Conrad MD, et al. Evolution of partial resistance to Artemisinins in malaria parasites in Uganda. N Engl J Med. 2023;389(8):722-732.
de la Fuente IM, Benito MJS, Ousley J, de Gisbert FB, García L, González V, et al. Screening for K13-propeller mutations associated with artemisinin resistance in Plasmodium falciparum in Yambio County (Western Equatoria State), South Sudan. Am J Trop Med Hyg. 2023;109:1072–6.
Dhorda M, Amaratunga C, Dondorp AM. Artemisinin and multidrug-resistant Plasmodium falciparum - a threat for malaria control and elimination. Curr Opin Infect Dis. 2021 Oct 1;34(5):432-439.
Dondorp, A.M. et al., 2009. Artemisinin resistance in Plasmodium falciparum malaria. N Engl J Med, 361(5), pp.455–467.
Emiru T, Getachew D, Murphy M, Sedda L, Ejigu LA, Bulto MG, et al. Evidence for a role of Anopheles stephensi in the spread of drug- and diagnosis-resistant malaria in Africa. Nat Med. 2023;29:3203–11.
Fola AA, et al. Plasmodium falciparum resistant to artemisinin and diagnostics have emerged in Ethiopia. Nat Microbiol. 2023;8:1911–9.
Jalei AA, Na-Bangchang K, Muhamad P, Chaijaroenkul W. Monitoring antimalarial drug-resistance markers in Somalia. Parasites Hosts Dis. 2023;61:78–83.
Juliano JJ, Giesbrecht DJ, Simkin A, et al. Country wide surveillance reveals prevalent artemisinin partial resistance mutations with evidence for multiple origins and expansion of high level sulfadoxine-pyrimethamine resistance mutations in northwest Tanzania. medRxiv. 2023 Nov 8 (pre-print) https://doi.org/10.1101/2023.11.07.23298207
Mihreteab S, Platon L, Berhane A, Stokes BH, Warsame M, Campagne P, et al. Increasing prevalence of artemisinin-resistant HRP2-negative malaria in Eritrea. N Engl J Med. 2023;389:1191–202.
Mohamed AO, Hussien M, Mohamed A, Suliman A, Elkando NS, Abdelbagi H, et al. Assessment of Plasmodium falciparum drug resistance molecular markers from the Blue Nile State Southeast Sudan. Malar J. 2020;19:78.
Moser KA, Madebe RA, Aydemir O, Chiduo MG, Mandara CI, Rumisha SF, et al. Describing the current status of Plasmodium falciparum population structure and drug resistance within mainland Tanzania using molecular inversion probes. Mol Ecol. 2021;30:100–13.
Ndwiga L, Kimenyi KM, Wamae K et al. A review of the frequencies of Plasmodium falciparum Kelch 13 artemisinin resistance mutations in Africa. Int J Parasitol Drugs Drug Resist. 2021; 16: 155-1
Owoloye A, Olufemi M, Idowu ET, Oyebola KM. Prevalence of potential mediators of artemisinin resistance in African isolates of Plasmodium falciparum. Malar J. 2021;20:451.
Peto TJ, et al. Triple therapy with artemether-lumefantrine plus amodiaquine versus artemether-lumefantrine alone for artemisinin-resistant, uncomplicated falciparum malaria: an open-label, randomised, multicentre trial. Lancet Infect Dis. 2022 Jun;22(6):867-878. Erratum in: Lancet Infect Dis. 2022 May;22(5):e128.
Phuc B, et al. Treatment failure of Dihydroartemisinin/Piperaquine for Plasmodium falciparum malaria, Vietnam. Emerg Infect Dis. 2017;23(4):715-717.
Phyo AP, et al. Declining efficacy of Artemisinin Combination Therapy against P. falciparum malaria on the Thai-Myanmar border (2003-2013): The role of parasite genetic factors. Clin Infect Dis. 2016 Sep 15;63(6):784-791.
Rosenthal PJ, et al. Cooperation in countering Artemisinin resistance in Africa: Learning from COVID-19. The American Journal of Tropical Medicine and Hygiene. 2022;106(6):1568-1570.
Rosenthal, P.J., Asua, V. & Conrad, M.D. Emergence, transmission dynamics and mechanisms of artemisinin partial resistance in malaria parasites in Africa. Nat Rev Microbiol 22, 373–384 (2024).
Spring MD, et al. Dihydroartemisinin-piperaquine failure associated with a triple mutant including kelch13 C580Y in Cambodia: an observational cohort study. Lancet Infect Dis. 2015;15:683–91.
Straimer J, Gandhi P, Renner KC, Schmitt EK. High prevalence of Plasmodium falciparum K13 mutations in Rwanda is associated with slow parasite clearance after treatment with Artemether-Lumefantrine. J Infect Dis. 2022;225(8):1411-1414.
Uwimana A, et al. Emergence and clonal expansion of in vitro artemisinin-resistant Plasmodium falciparum kelch13 R561H mutant parasites in Rwanda. Nat Med. 2020;26:1602–8.
Uwimana A, Umulisa N, Venkatesan M et al. Association of Plasmodium falciparum kelch13 R561H genotypes with delayed parasite clearance in Rwanda: an open-label, single-arm, multicentre, therapeutic efficacy study. Lancet Infect Dis. 2021; 21: 1120-1128
van der Pluijm RW, et al. Determinants of dihydroartemisinin-piperaquine treatment failure in Plasmodium falciparum malaria in Cambodia, Thailand, and Vietnam: a prospective clinical, pharmacological, and genetic study. Lancet Infect Dis. 2019 Sep;19(9):952-961.
World Health Organization (WHO), 2022a. Accelerating malaria elimination in the Greater Mekong. The Mekong Malaria Elimination programme, Bulletin 10, March 2022 Geneva.
World Health Organization (WHO) 2022b. Strategy to respond to antimalarial drug resistance in Africa. Geneva: World Health Organization; 2022. Licence: CC BY-NC-SA 3.0 IGO.
Previous WWARN K13 Genotype-Phenotype Study Group Publication
WWARN K13 Genotype-Phenotype Study Group. Association of mutations in the Plasmodium falciparum Kelch13 gene (Pf3D7_1343700) with parasite clearance rates after artemisinin-based treatments—a WWARN individual patient data meta-analysis. BMC Medicine. January 17, 2019.